Category: Sin categorizar
Breakthroughs While Breaking Ground: The First TBM Conveyor
Modern Achievements
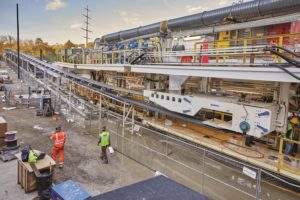
The 100th conveyor system has been refurbished and customized for the job in a process that Robbins has been perfecting for decades.
There are many elements that contribute to the success of a project: having an accurate report of ground conditions, choosing the proper equipment, having a well-trained team operating the tunnel boring machine—and behind it all—the utilization of a high-quality conveyor system. As tunneling machines evolve and bore at continuously faster rates, rapid muck removal becomes more important than ever. Robbins conveyor systems are capable of moving more than two thousand US tons of muck per hour—saving time and money on every project that uses the complete system.
In Akron, Ohio, a major milestone has been reached for Robbins conveyors. Running behind a Robbins 9.26 m (30.4 ft) diameter Crossover TBM is the 100th Robbins Continuous Conveyor System supplied for muck removal—more than any other TBM conveyor supplier has sold. The conveyor in Akron is just one landmark event in the long history of Robbins conveyors, the start of which can be traced back to the first ever documented use of a continuous conveyor system behind a TBM.
Pioneering Muck Removal
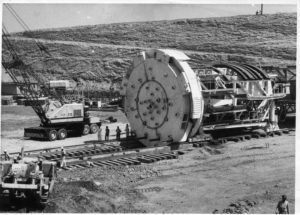
No roads were at the site in West Pakistan so goods were transported by rail car, requiring surveying of hundreds of bridges from the port city of Karachi.
The prototype for the conveyor systems we know today was developed by James S. Robbins in 1963 and was built using a Goodman coal-mine conveyor instead of muck cars which were more commonly used during that period. The conveyor ran behind what was, at the time, the largest TBM in the world. The 11.2 m (36.7 ft) diameter Robbins Main Beam was built for the Mangla Dam Project in what was then known as West Pakistan. The 4.3 km (2.7 mi) long tunnel was built to control water flow from the Jhelum River for use in agriculture and hydro-electric power.
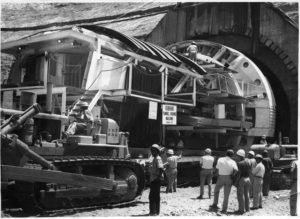
Temperatures at the jobsite climbed up to 53 degrees C (127 degrees F) in the shade.
The conveyor design had originally been used for coal and potash mines and was adapted for use behind the TBM. An extendable belt was side-mounted in the tunnel, leaving room for man-access and rail tracks to carry materials. Belt tension was maintained using a belt cassette. “They were able to operate continuously. It worked like a charm—and then the extensible conveyor was forgotten by the industry because it was perceived by contractors and owners as too much of an investment,” explained Dick Robbins, former President and CEO of The Robbins Company, in a 2013 article for World Tunnelling. It would be another 30 years after the successful completion of the Mangla Dam tunnel until conveyors became a standard means of muck removal in the tunneling industry.
Persistently Moving Forward
Conveyors have come a long way since their first use. Unlike the system used for the Mangla Dam project—which was limited to the use of a straight belt—conveyors can now wrap around curves and bring rock vertically up a shaft to the surface. “The control system, along with monitoring systems, has dramatically improved,” says Dean Workman, Robbins Director of Conveyors, Cutters, and SBUs. “The components have all been enhanced, giving a much longer life to the conveyor system. Contractors are using the same conveyors on multiple projects with minor refurbishments between projects.” While the basic function of a conveyor has stayed the same—muck hauling—the quality of conveyor systems has certainly changed.
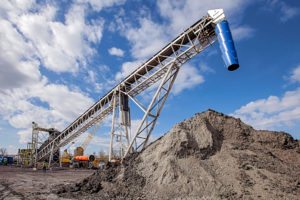
Did You Know: At least 75% of all world records achieved by TBMs were done with a continuous conveyor system for spoils removal rather than muck cars.
In a new design that is being used for the Los Angeles Purple Line Extension, continuous conveyors have gained another ability, they are now able to change direction. The unique system can be flipped to accommodate two machines boring parallel tunnels eastward before being re-launched to bore west. Due to the narrow launch shaft, modular components were designed to save space, and were built using a kit of secondhand parts, showcasing the long-life conveyor systems are capable of. “We are always looking to improve the design and function of the system,” says Workman, “modular components are a benefit to the contractor when installing, maintaining, and shipping the equipment.”
As progress continues and new technology provides advancements for the industry, The Robbins Company continues to uphold the pioneering spirit that James S. Robbins brought to tunneling with his trailblazing developments.
Read More:
Robbins Conveyor Systems
Conveyor Spec Sheet
100th Conveyor
What's Going On? A Quarterly Worldwide Jobsite Roundup
At any given time, Robbins TBMs are operating at dozens of jobsites around the world. Our dedicated Field Service personnel take video and pictures of the TBM progress often, so we’ve decided to offer a quarterly roundup of what’s going on in picture and video format–from deep TBM assembly in New York, USA to an epic TBM launch in the Himalayan mountains of Nepal. Read on to found out the latest.
TBM Assembly in a Deep Shaft in New York, USA
A 6.8 m (22.3 ft) Robbins Single Shield TBM, designed for water pressures up to 20 bar, is undergoing assembly and testing at the Delaware Aqueduct Repair Project. The TBM will be launched from a starter tunnel at the bottom of a 274 m (900 ft) deep shaft.
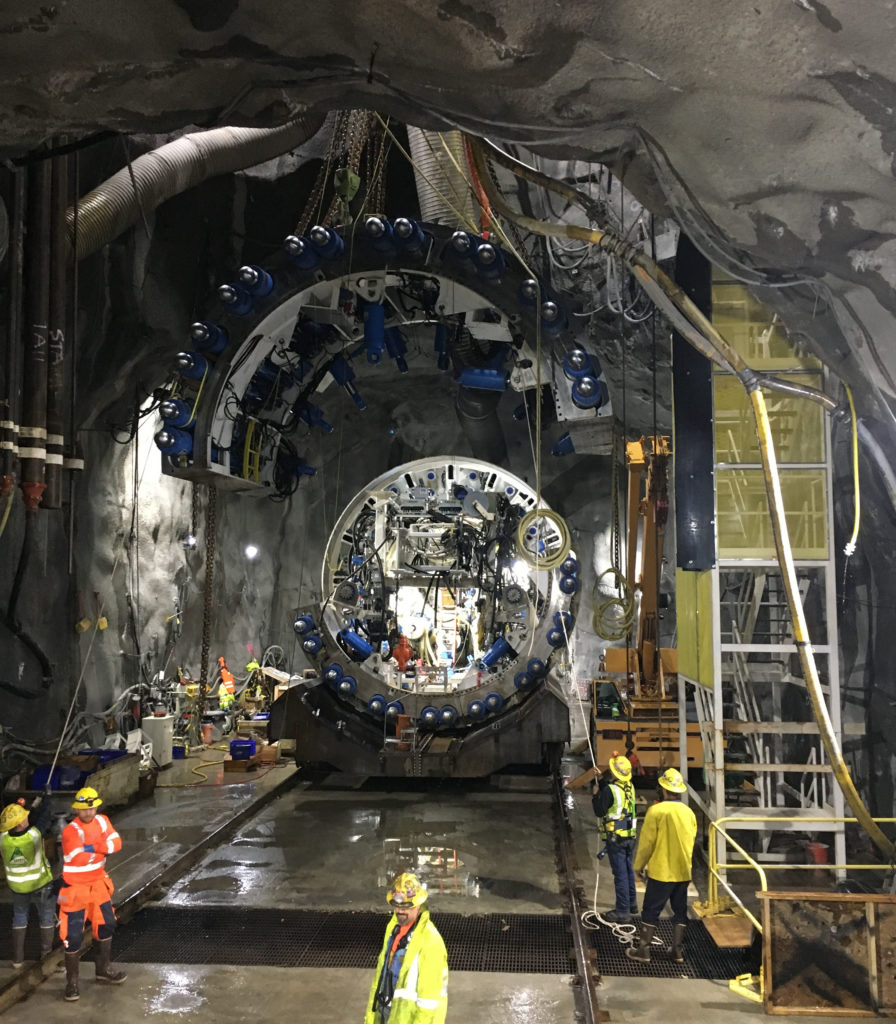
TBM Assembly at the Delaware Aqueduct Repair in Newburgh, New York, USA. The shaft is an incredible 274 m (900 ft) deep.
TBM Assembly Time Lapse
Epic TBM Launch in the Himalayan Mountains
In the Siwalik Range of the southern Himalayan Mountains of Nepal, a 5.06 m (16.6 ft) Robbins Double Shield embarked to bore the Bheri Babai Diversion Multipurpose Project (BBDMP). The TBM launched on October 14. See the drone footage taken by our own Field Service TBM Mechanic Thomas Fuchs:
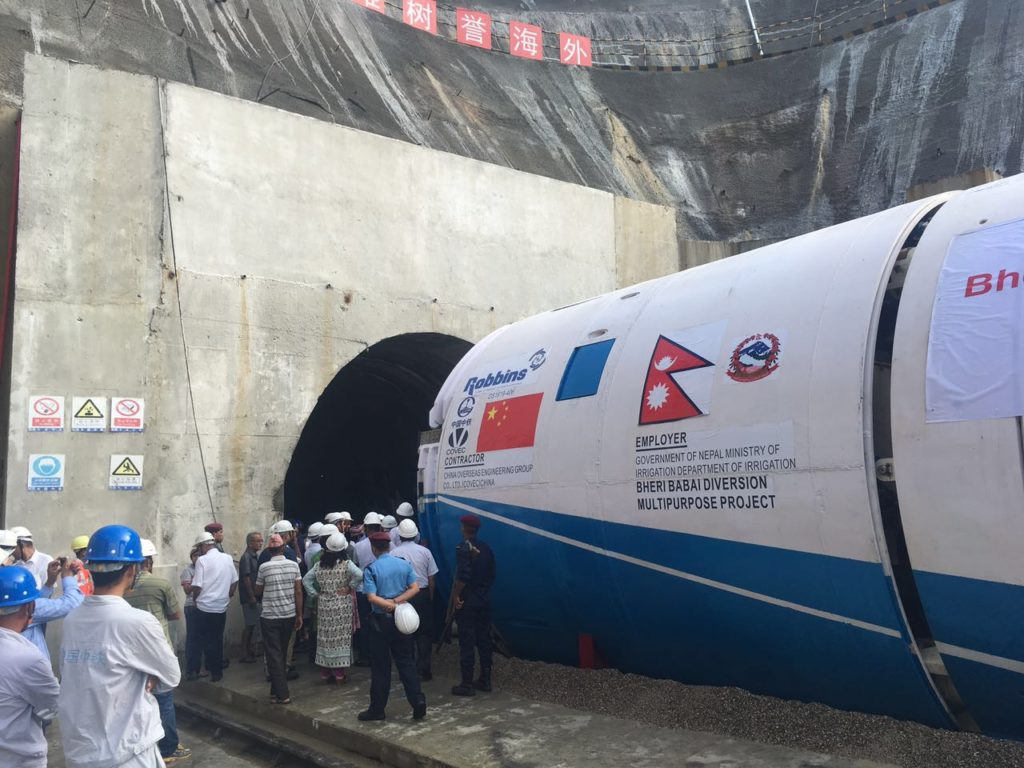
Launch of the Robbins Double Shield at Nepal’s BBDMP on October 14.
Crossover Machine Startup in Akron, Ohio
On October 19, a Robbins 9.26 m (30.4 ft) diameter Crossover (XRE) TBM launched below Akron, Ohio, USA to bore the OCIT tunnel. The machine is excavating in soft soils that will transition into mixed face and then full face shale. Before its launch, personnel at the jobsite filmed the cutterhead testing in a unique way. Watch the video below for more:
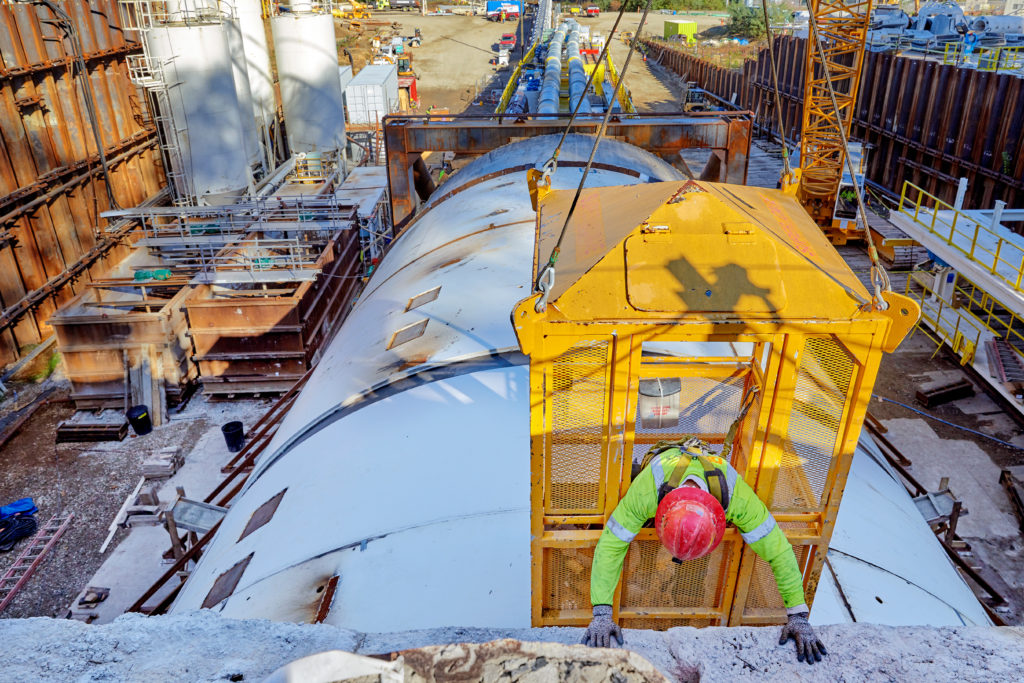
A worker surveys the TBM prior to its launch on the Akron OCIT October 19.
Boring the Blue Mountains: The Inside Story on the World’s Fastest TBM
Over 20 years ago, a Robbins open-type machine set three world records while tunneling in the picturesque Blue Mountains in Australia. You may be asking yourself, why is this significant? Why drudge up a project that is surely outdated at this point in our industry’s history? The fact of the matter is, two decades have passed and the Robbins open-type TBM chosen for this project is still considered to be the world’s fastest TBM.
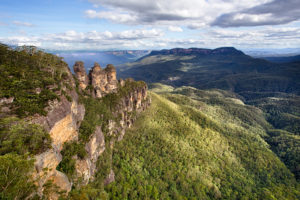
The picturesque Blue Mountains in New South Wales, Australia.
Project Background
In 1993, the 3.4 m (11 ft) diameter TBM was chosen to bore two sewage tunnels in the Blue Mountains near Sydney, Australia. At the time, there had been a rapid expansion of urban developments within the Blue Mountain National Park, causing an influx of pollution to enter streams as the result of septic tank runoff and outdated sewage treatment plants.
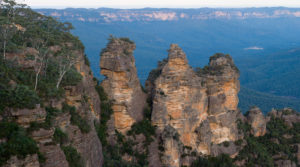
A closer look at the Three Sisters, a unique rock formation in the Blue Mountains.
The Blue Mountains Sewage Transfer Project comprised of approximately 40 km (25 mi) of tunnels, two of which were TBM-driven using the Robbins machine. The first, the Katoomba Carrier tunnel, was 13.4 km (8.3 mi) long and the second, the Lawson Carrier, was 3.5 km (2.1 mi) long. While the Lawson Carrier tunnel was finished five weeks prior to the expected completion date, it was during the Katoomba Carrier tunnel that all three records were set. During excavation the machine set the following world records: best day of 172.4 m (565.6 ft), best week of 702.8 m (2,305.7 ft), and a best monthly average of 1,189 m (39,000 ft) within its size range of 3 to 4 m (9.8 to 13.1 ft) diameter machine. Two of those records—the best day and best week—are the fastest ever recorded and have yet to be surpassed by any TBM of any size.
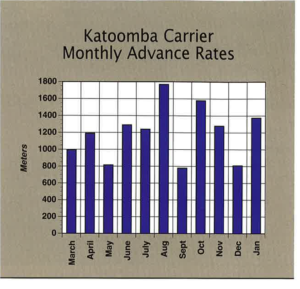
The 3.4 m (11 ft) diameter TBM set three world records while boring the Katoomba Carrier tunnel. Photo credit: tunneltalk.com
Custom Machine Design
There were many factors that played into this machine’s success—the system utilized on this project required a well-planned design, careful operation, and regular maintenance. As many people involved in tunneling know, choosing the right equipment for the geology can make or break a project. Detailed empirical data allowed the contractor to accurately predict what kind of ground they would encounter and prepare accordingly. The National Park is located within the Triassic Sydney Sedimentary Basin and is comprised primarily of sandstones and claystones. Anticipating this geology, the 3.4 m (11 ft) diameter machine’s cutterhead was dressed with 25 Robbins 17-inch diameter disc cutters designed for soft yet abrasive rock formations.
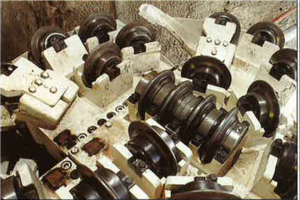
A close-up of the 17-inch cutters.
Logistics
The 13.4 km (8.3 mi) long Katoomba Carrier tunnel was originally planned to comprise of multiple short tunnels, several kilometers in length each, with intermittent portals to shorten drives. Ultimately it was decided to make the project one continuous tunnel, which led to higher advance rates and deemed it—at the time—the longest single-drive TBM tunnel. Not only did this change save time, but it also allowed boring to be less disruptive to the landscape.
Continuous Conveyor
In addition, the project was the first in Australia to utilize a continuous conveyor system. Due to the length of the tunnel, the use of traditional muck cars for muck removal would have taken too much time and were seen as an inadequate solution. The 106-m (347.8 ft) long system boosted production rates, with a best day of 1,565 m³ (55,267 ft³) of in-situ material removed from the Katoomba tunnel.
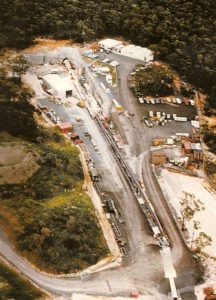
The Blue Mountains project was the first TBM-driven tunnel in Australia to utilize a continuous conveyor system.
Good Geology
Ground support throughout the tunnel comprised of a combination of resin grouted bolts, mesh, steel straps and steel sets. In the sturdier sandstone, however, the tunnel was left largely unlined and instead shotcrete was applied to areas of poorer, softer rock.
As boring progressed, the sandstone proved to be softer than expected. Instead of the predicted average of 80 MPa ranging from 20 MPa to 150 MPa, it averaged 40 MPa to 50 MPa with a range of 10 MPa to 100 MPa. This ideal material could also have contributed to the Robbins machine’s record breaking results and its early breakthrough, which occurred nine months ahead of schedule.
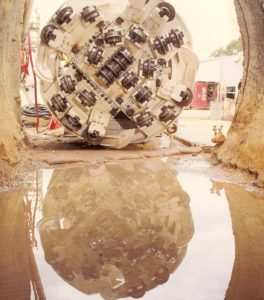
The machine’s record breaking boring enabled the project as a whole to finish 17 months ahead of schedule.
Exceeding Expectations
The Robbins Mk 12C’s performance on the tunnel boring portion of the project substantially surpassed all expectations. Not only did it set the previously mentioned world tunneling records, but it also helped the project as a whole finish 17 months ahead of schedule, saving not only on time but on significant financial costs.
The Changing Face of the Tunneling Industry: The Role of the ITA, Partnership, and Innovation
Martin Knights graduated with a degree in Civil Engineering in 1970 and joined the Second River Mersey Road tunnel project as a site engineer in Liverpool, UK as his first TBM project. The major feature of this 2.5 mile long twin tunnel project was the refurbished 35 ft diameter Robbins TBM, which had previously driven a number of water supply tunnels at the Mangla Dam in Pakistan. Knights worked in several consultancy firms on a number of high profile tunnelling projects over several decades, most recently as the Managing Director and Sr. Vice President for CH2M/Halcrow. He was also president of the International Tunnelling Association (ITA) from 2007-2010. He is currently an independent consultant with his own company, Martin Knights Consulting.
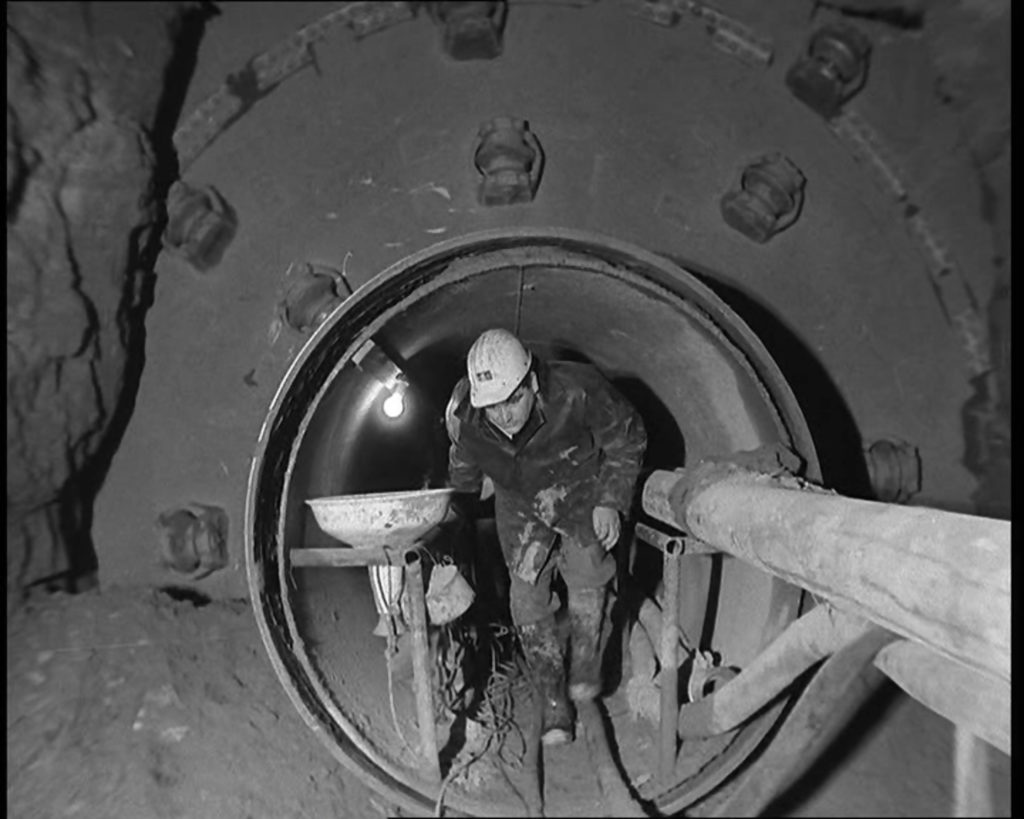
A worker exits the rebuilt Robbins TBM used on the Mersey Road Tunnel Project in the UK. The Second Mersey Road Tunnel was Knights’ first project after graduating in 1970. Image Credit: British Pathé
At the recent ITA Training Course in Bergen, Norway, which preceded the World Tunnel Congress, I gave a lecture about the development of Soft Ground TBMs from Victorian times until now. It took a bit of research: I trawled through reference books and talked to experts in the TBM world. During the research I looked at project specifications for big projects carried out in the past 40 or so years, and was struck by the level of technical detail and requirements that engineers stipulated in the contract documents: there seemed to be a tendency for over-specification that imprisoned the contractor and his equipment suppliers to past tried-and-tested practices. Where was the opportunity to innovate and trial new ideas by those best suited to introduce best and new practices?
That reminded me of Lok Home’s [Robbins president] challenge to me in November 2009 at the Hamburg, Germany STUVA Exhibition and Conference. Lok and l, and other colleagues in ITA and the tunnelling world, took part in an industry round table talk. I was President of ITA then and the two-hour discussion concluded with Lok asking me, “So, Martin, what can I and the industry do for ITA? We provide sponsorship to ITA, like other leading tunnelling companies; we fill the exhibition halls; we host social gatherings during ITA conferences, and we give lectures promoting new products. But we as equipment manufacturers and suppliers want to play a bigger technical role.”
Stung by the realisation that ITA had, by default, missed a trick, and was, in effect, excluding the very talent that provided innovation in tunnelling, I set about forming the then-new ITA technical forum ITAtech. I say “I”, but in fact myself, Lok, Tom Melbye of Normet, Felix Amberg of Amberg Engineering, and colleagues from Herrenknecht, Mapei, Atlas Copco, and others set out over three months to prepare the ground to launch ITAtech as a premier promoter within ITA of technology and innovation. Our purpose was to prepare independent and commonly-agreed technical guidelines and evidence endorsed by the whole international tunnelling industry, which is what ITAtech continues to do. It provides manufacturing, installation, equipment and materials guidelines for Contractors and Designers and creates confidence for tunnel owners that the “brand” of the ITA is overseeing this important knowledge transfer.
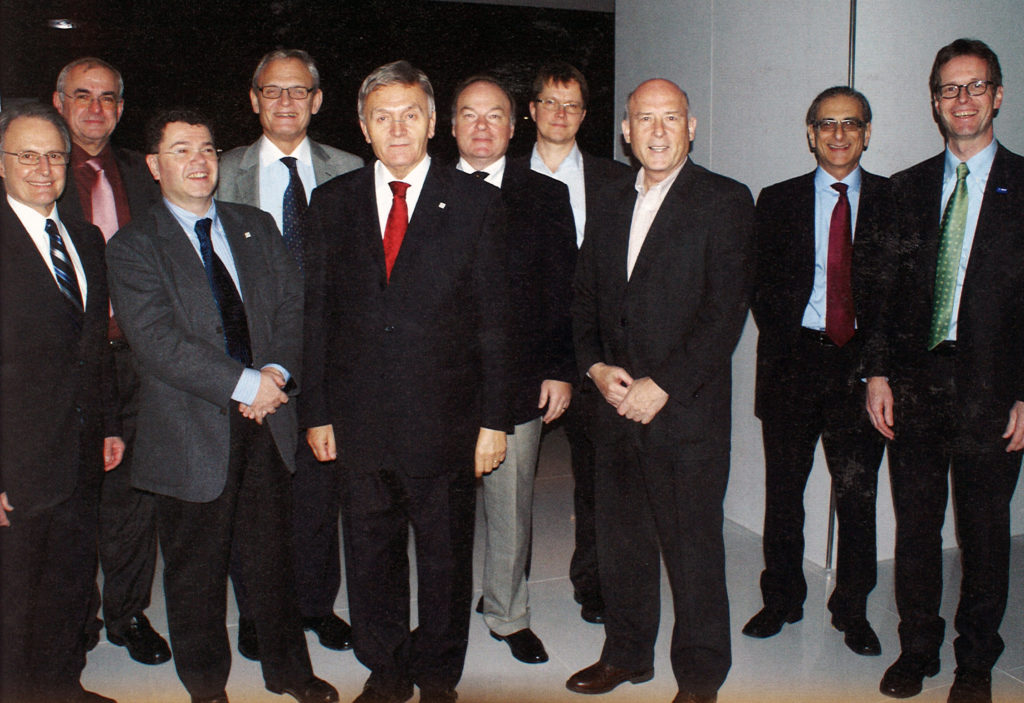
The initial 2009 meeting that led to the ITAtech forum. Left to right: Bill Hansmire, Gerhard Robeller, Olivier Vion, Gunnar Nord, Martin Knights, Brian Fulcher, Pekka Nieminen, Lok Home, David Caiden, Daniel Ruckstuhl
As I talked to the Bergen delegates this year, it was obvious to me that soft ground urban tunnelling owes a debt to the development of TBMs over the past 40 years…and particularly to the past 10 years. We can now do things that were too difficult to do way back then. It’s no coincidence that the innovations in the last 50 years were led by TBM companies like Robbins, Lovat, Seli, Herrenknecht, etc. The leadership and pioneering spirit could have only been driven by the individual passion that comes from a “family run” business. With time, growth and ownership has been passed on, but the stewardship and spirit of innovation in tunnelling lives on. ITAtech has tried to capture that spirit. Membership of its Steering Board and Activity groups relies on that desire of industry to educate, improve and share.
A recent President of the UK Institution of Civil Engineers compared our fragmented industry processes with the manufacturing, aircraft and car industry. In effect he said “Why–unlike those industries–do we separate inception, planning, and design from assembly, installation and construction?” The infrastructure and mining industries rely on so many processes and partnerships to deliver projects precisely because they are so fragmented and siloed.
Contemporary procurement encourages more partnerships, but this doesn’t make it any less convoluted. We see the rise of the dreaded words “industry supply chain” and “second and third tier partners”, where it strikes me that we’re airbrushing out the identity of the very “tier” of industry that has to innovate and provide the substance that nourishes the technical solutions that feature in new infrastructure developments.
I suggest that owners and clients get better access to and value from these innovating “tiers”—in many cases the equipment manufacturers and suppliers. In so many cases I have seen technical solutions get misinterpreted as ideas and solutions move up and down the so-called supply chain, begging for recognition, understanding and realisation of value.
I’m sure that this is not intended…but it happens. By validating and bringing innovations to the forefront through organizations like the ITAtech, we can improve some of the convoluted procurement and technical approval processes. However if we want to continue to see advancements like we’ve seen in urban soft ground tunnelling in recent decades, we should seek to streamline manufacturing and procurement, and to work closely with all “tiers” involved—second, third, and on up.
5 Things DGS can do for your TBM Operation in Difficult Ground
Difficult Ground Solutions (DGS) is a suite of options that can be added to a shielded hard rock machine or Crossover machine to better enable advance when conditions are unknown or difficult conditions are anticipated. While each of these items is not new, the concept of installing them on a machine from the start, and of using them in concert to provide better visualization and performance in the most challenging ground, is. The uses and advantages of DGS are various and wide-reaching.
Number 1: DGS can act like risk insurance.
Yes, risk insurance. While one can argue that risk insurance is not needed—and of course if a tunnel were in entirely homogeneous, medium strength, self-supporting rock, under a medium amount of cover, I would tend to agree—there is a problem with this argument. That scenario exists only very rarely. At Robbins, we are seeing more tunnels being planned in mountainous, remote regions where an accurate GBR cannot be obtained. More tunnels planned through multiple fault zones and mixed face conditions. More tunnels planned in suspected karst conditions and in rock where large water inflows may occur. It is a trend we’ve observed arcing upward, and with these high-risk projects it seems prudent that an equipment manufacturer should strongly recommend risk insurance. We’ve done that the only way we know how: by calling on our extensive experience to develop, test, and provide various solutions that can be added to a TBM at the beginning of the project. These features mitigate the risk of getting stuck in unforeseen (or foreseen) challenges that might come up in a tunnel drive.
This risk insurance works like any other type of insurance—it must be purchased before the actual event happens. Waiting until a machine encounters a given challenge—where it may become damaged, stuck, or irretrievable—is not an adequate plan for difficult conditions. Sure, some of these options can be added in the tunnel, but such an operation is far from ideal. Risk insurance is best purchased during the TBM design phase, long before the machine is built and launched.
Number 2: DGS can get your TBM through a fault zone or squeezing ground where it might otherwise become stuck.
If fault zones or squeezing ground are known or suspected, or if there is even a possibility of encountering them, this can greatly affect your TBM operation. Shielded hard rock machines protect your crew from the surrounding ground, and they can bore and line a tunnel efficiently, but in fault zones and converging material they need some of the features of an EPB machine to keep advancing. Avoiding a stuck machine is paramount to project success. Luckily, DGS uses several ways to avoid the problem of a machine becoming stuck. The first of these is multi-speed cutterhead drives. These drives effectively give the machine multiple modes of operation—high speed, low torque for hard rock, and low speed, high torque for difficult ground. Designing a machine with high-torque, continuous boring capabilities allows that machine’s cutterhead to restart with break-out torque in difficult ground. The net effect is that the machine can keep boring in the event of a face collapse and can effectively bore through fault zones and running ground where the potential for cutterhead jamming exists. Going one step further, multi-speed gearboxes give the machine the ideal EPB-type torque if larger sections of soft ground are anticipated.
Secondly, TBMs can get through squeezing ground and faults using Continuous Shield Advance. This design utilizes a stepped shield configuration—where each successive shield is slightly smaller in diameter—to avoid becoming stuck in converging ground. External shield lubrication is an added insurance against becoming stuck, using a series of radial ports that can pump Bentonite into the annular space to act as a lubricant in squeezing material.
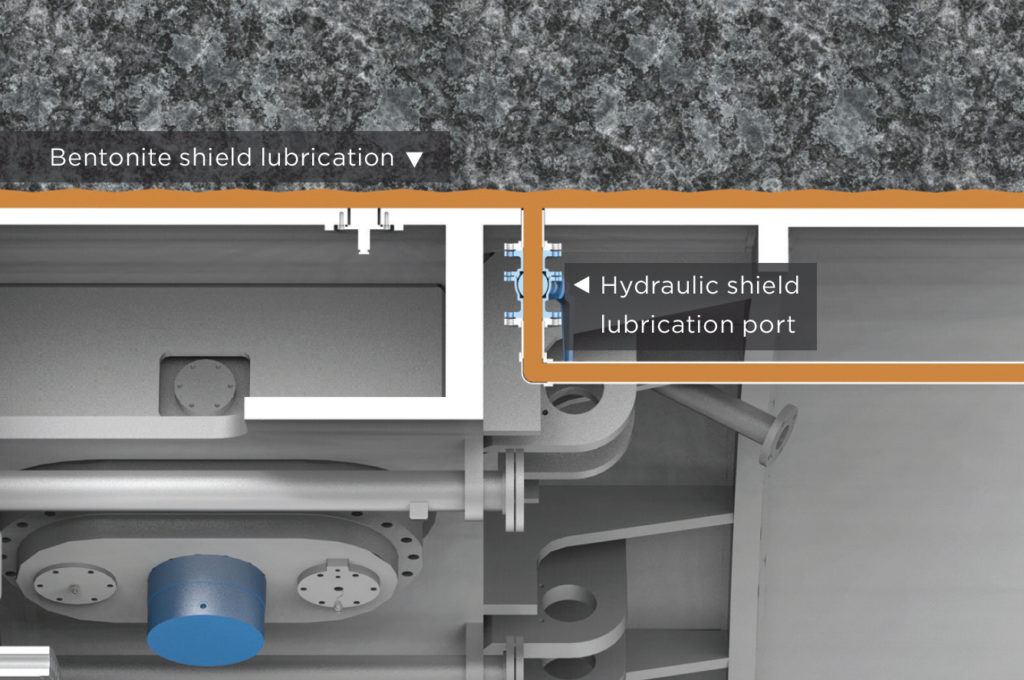
Hydraulic shield lubrication allows for Bentonite (orange) to be pumped into the annular gap.
As a last effort, if a machine has already become stuck the TBM can utilize augmented, or “super” thrust. Additional thrust jacks can be added to supply an extra boost in a short stroke, generating enough force to break loose a trapped shield.
Number 3: DGS can keep your crew safe and save your TBM in the event of a massive inrush of water.
Water is an ever-present part of tunneling underground, but unexpected large inflows can damage a machine and grind a TBM operation to a halt.
In the event of a large inrush of water, a guillotine gate on the muck chute can effectively seal off the muck chamber of a Single Shield TBM to keep the crew safe as well as keep the machine from becoming flooded out. This system is termed “passive” water protection because the TBM is stopped in place (not actively operating). During that time the crew can then work to grout off water inflows and dewater the chamber to control the flow before they begin boring again. The grouting crew also have the added assistance of back pressure to assist in grouting.
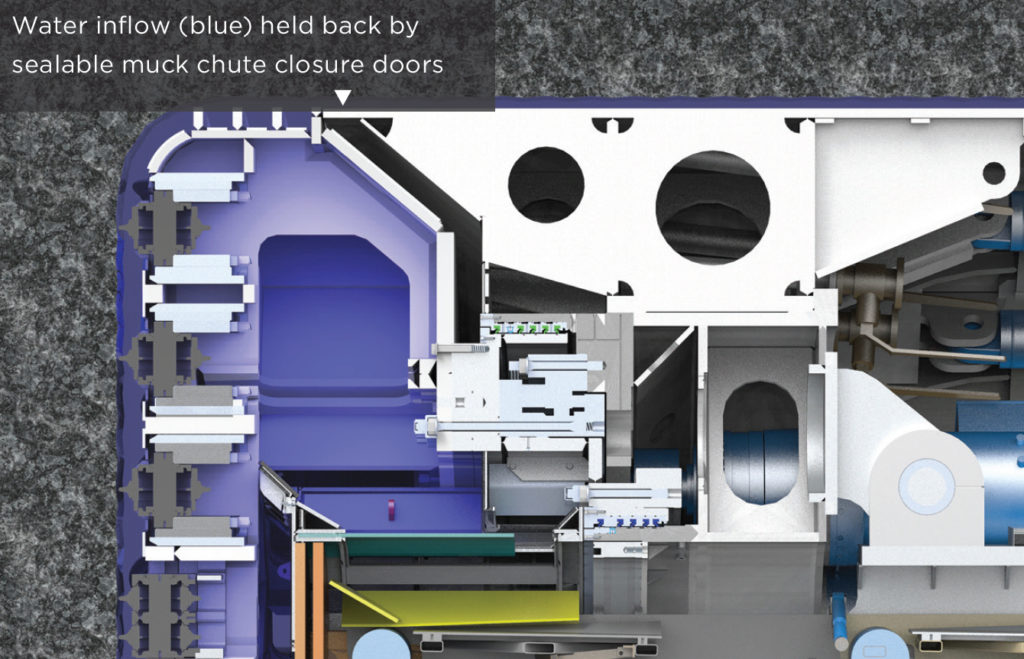
Water Inflow Control can passively seal a Single Shield TBM against water (blue) via a guillotine gate.
Number 4: DGS can improve your visualization of the ground around the TBM.
Probe drilling is an essential part of visualization, and, combined with grouting, it can also fall under the heading of water control. We’ve learned that, in difficult ground, more is better. Multiple probe drills, and more drill ports in a 360-degree radius, are always going to give an advantage. We’ve taken this lesson to heart, and have installed multiple probe drills on many of our new shielded machines, with ports to provide probing patterns in a 360-degree radius. High-pressure grout injection can be done through these same ports to stabilize ground up to 40 m ahead of the face (or more if using specialized drills). The type of grout injected can also be specialized—for example chemical or polymer grout can be used to seal off groundwater. Lastly, a rotary forepole drill can be installed behind the cutterhead support to allow for ground consolidation around the shield periphery. The forepole drill is of particular use in fractured rock and fault zones. These drills are the mainframe of a visualization plan that can ultimately create an in-tunnel GBR as the machine advances: of particular use when no accurate GBR can be created due to topography, high cover, etc.
But what if you suspect the ground around your TBM is converging? What if you want to get a look at the actual rock face in the safest possible manner? There are DGS options for these types of visualization as well. For squeezing ground detection, a hydraulic cylinder can be mounted on top of the shield and connected to the TBM’s PLC. It measures the shield gap in the tunnel crown, so that if squeezing or collapsing ground is detected the crew can take countermeasures. These measures include using bentonite lubrication, crown or face rock conditioning, or planning ahead to use another system in the area before the machine can become stuck. For getting a better look at conditions ahead of the machine, a cutterhead inspection camera can be used to remotely inspect the boring cavity without intervention, and to check water levels ahead of the TBM. While these cameras have been used to monitor mixing chambers and perform cutterhead inspections in soft ground TBMs, their use in hard rock machines has been much more limited. In the new ground investigation system, the probe and injection holes in the cutterhead and front shield are specifically designed to accept these cameras.
Number 5: DGS can save you time and money.
The old adage “the best-laid plans can go astray” applies particularly well to tunneling. There are many unknowns, even with an adequate GBR in hand. Making an initial investment on DGS features when your TBM is still in the shop is far less costly than installing them in the tunnel after a major stoppage. These features can mean the difference between a successful operation and a stuck TBM requiring a bypass tunnel or worse. TBMs with DGS features also produce better advance rates in adverse conditions such as fault zones (see our recent projects Kargi and TEP II as good examples of this). Better advance rates mean your project is more likely to stay on schedule and on budget. And who wouldn’t want that?
Want to find out more about DGS and its successful use in the field?
Check out these white papers:
Difficult Ground Solutions: New TBM Solutions carve a path to Success
What’s in a Name? Mixshield, Crossover, Hybrid and More
For underground construction professionals and manufacturers, there is some confusion about the current TBM designations and what they really mean, and for what applications they are to be considered. This is especially noted when it comes to soft and mixed ground type shield machines and if they are to operate in “open” mode, “closed” mode or “semi-open/closed” mode conditions.
The term “open mode” defines defines a mode of TBM operation where no pressure is required to stabilize the boring face; the geology is self-standing and ground settlement will not occur.
The term “closed mode” defines tha mode of TBM operation where face pressure needs to be constantly applied in order to maintain face support. Should the face pressure not be maintained as determined by the geology, under-pressurization will cause ground settlement, over-pressurization can cause surface heaving.
The term “semi-open/closed mode” defines a mode of TBM operation in varying geology where the TBM has the ability to control the face.
The various machine types in question are Mixshield, Hybrid and the Robbins “Crossover”. Are they the same? If not, what is the difference? This blog seeks to clarify and explore those definitions.
Mixshield
Mixshield is a terminology introduced by German manufacturer Herrenknecht for what is essentially a Slurry TBM that uses an air bubble to control and support pressure at the face. The air bubble acts like an accumulator with the belief that this offers better pressure control. This type of machine operates only in “closed mode”. Mixshields are not meant to go from high pressure soft ground to significant rocky conditions with great efficiency, hence the name “Mixshield” is somewhat of a misnomer. They can excavate mixed face conditions, but the machine configuration is not changed so it is a different thing entirely than to excavate significant sections of differing geology. In order to change the configuration to allow more efficient operation in different sections of geology, a Hybrid machine is needed.
Hybrid, etc.
“Hybrid”, “Dual Mode”, and “Multi-Mode” are names developed by the industry to try and define machines that feature components of two different machine types. The most common types is a multi-mode between EPB with a screw conveyor (for operation in “closed mode” or “semi-closed mode”) and conversion to Rock Mode with the installation of a belt conveyor (for use in “open mode” operation). Typically, the only changes made to the machine are components such as cutting tools and muck removal method that can be switched out in the tunnel depending on ground conditions. The machines are used for large sections of vastly different geology in one tunnel, such as a section of clay followed by as significant section of rock. However these types of machines are typically designed as EPB machines and then converted, making them not very efficient for use in rock geology.
Robbins Crossover
The “Crossover Series” of TBMs was developed by Robbins in March 2015 using innovative design concepts to effectively excavate between different geologies. The Crossover Series is Robbins’ version of a Hybrid TBM but with many additional features to allow the TBM to effectively cross between different geology types. Operation of the machine can allow the machine to quickly change from “open mode” to “semi open/closed mode” and “closed mode.” The main types of Crossover TBM include the XRE (Crossover between Hard Rock and EPB), XSE (Crossover between Slurry and EPB) and XRS (Crossover between Hard Rock and Slurry). The features include interchangeable cutting tools; single direction and bi-directional cutterhead muck pick-up; multi-speed, multi-torque cutterhead drive systems; durable, abrasion resistant components on the cutterhead and screw conveyor; emergency thrust; tapered shields to reduce shield entrapment in squeezing ground; integrated probe/grout drill system for ground consolidation; and heavy steel construction with components designed for +12,000 hours of life, just to name a few.
The Crossover Series is suitable for tunnel projects that require the ability to excavate different types of geology efficiently with one machine, based on ground conditions. The TBM design is flexible enough to switch from one mode to another. Ultimately, Crossover Series machines are cost efficient in the long run, since the TBMs require less repair costs than a non-customized machine, and they can be used on multiple projects.
Based on the ground situation (whether it’s self-supporting ground, self-supporting ground with water pressure, or unstable ground), the Crossover is optimized towards “open” mode (as in hard rock shielded TBMs) or “closed” mode (as in compressed air, slurry, and EPB TBMs).
Reliable geological information is therefore critical to the TBM design. An accurate geological report is needed to decide when and where to convert the machine as well. During excavation, adequate probe drilling is further essential to determine the ground conditions ahead of the Crossover TBM.
Below are the features specific to each type of Crossover machine.
Crossover XRE
- Mixed ground cutterhead: with disc cutters & soft ground tools or a combination of tools (interchangeable).
- Two-speed gearboxes: provide high torque at low RPM under soft ground conditions and high RPM under hard rock conditions.
- Cutterhead rotation: Single direction is more efficient in hard ground conditions and bi-directional is more efficient in soft ground to prevent roll.
Crossover XSE
- Equipped with both screw conveyor and slurry system for muck removal.
- Ground conditions: soft ground containing water under pressure (particularly for water pressures > 5 bar).
- The most universal of the Crossover machines, the XSE can bore in most types of ground.
Crossover XRS
- Highly adaptable to variable ground conditions; suitable for rock tunnels with water pressure > 5 bar.
- Hard rock machine with a rock cutterhead and slurry system in place.
- Capable of mining rock through high water pressure without grouting off water flows.
Rebuilt, Refurbished, Remanufactured: Designing a TBM to Live for Generations
With the increase in large TBM projects over the last few decades and a global awareness of their environmental impacts, there has been a greater focus on the origin of TBMs and their parts. The focus has been further highlighted by the ITAtech; a technology-focused committee for the International Tunneling Association (ITA-AITES) that produced guidelines on rebuilds of machinery for mechanized tunnel excavation were released in 2015. While the guidelines are relatively new, Robbins has a long history of delivering robust machines, many of which are rebuilt (many are also 100% new). In this post, I’d like to explore just what a “rebuilt” TBM is, and what that means to Robbins as a TBM manufacturer.
History and Terminology
Throughout Robbins’ history (over 65 years), our TBMs and design philosophies have been based on the understanding that TBMs require a substantial initial investment, and designing machines for single tunnels is neither economical nor sustainable. This realization has resulted in robust, sturdy designs of high quality that–even before the pencil is put to paper–are meant to last for multiple projects. This is clearly shown when you look at the number of Robbins TBMs around the world that are excavating their second, third, fourth or even their eighth or ninth tunnel. There are even Robbins TBMs manufactured in the 1960s that are still in operation!
For Robbins, the term “rebuilt” describes any manner of creating a custom TBM from already existing components. It is the term we use most and continue to use. The ITAtech guidelines introduce different terminologies depending on the extent to which a TBM is rebuilt. They are, very shortly, described here:
- Remanufacturing – Remanufacturing is a process with the aim to start a new life cycle of the product using its current or modified configuration.
- Refurbishment– Refurbishment can be considered a full maintenance, where defect parts are replaced to extend the life of the product in its original configuration or with small modifications.
The guidelines describe the requirements of each process in order to designate a TBM “refurbished” or a “remanufactured”, but in reality the majority of “rebuilt” TBMs may be somewhere in between these qualifications.
The Robbins Philosophy
Over the years Robbins has built a quality assurance system that ensures when we deliver a rebuilt machine, either to the original configuration or a modified one, we still adhere to a design life of 10,000 hours. This standard also includes checks to make sure that all the components are in a functional condition of ‘as new’ or ‘new’. Due to our long experience in rebuilding TBMs, we can offer in principal the same warranty on a rebuilt machine as a new machine.
The Robbins philosophy on this is that, in order to offer the same design life and same warranties on a rebuilt machine, the initial design of the TBM will need to consider that the TBM will be used on several projects. This means that the major structures will need to be strong enough to survive even the toughest conditions and that worn parts can easily be replaced. If the machine is not properly designed for multiple projects, there will be a need to do major work to get the TBM in a working condition either in its original or modified configuration. Robbins strongly believes that considering the total life cycle of a machine, even in its first design stages, is the most economical and sustainable option.
One can argue that project owners typically only have one project and that the condition of the TBM and the suitability of its rebuild is therefore not essential. This is something that is also reflected in many of today’s tunneling projects, where the commercial consideration is often given far more attention than the technical one. We would argue, however, that an initially sturdy and robust design of the TBM will give the project more uptime, higher production rates and better flexibility if unexpected conditions are encountered, making it a good and effective kind of insurance for the project. This effect has been clearly identified in the field, where Robbins has more than 90% of world production records in hard rock.
What’s Missing
In terms of the international guidelines, they are certainly necessary and welcomed. However, the strictness of the guidelines makes them hard to adopt worldwide and perhaps not realistic for the majority of TBM rebuilds, which are customized based on project needs. The ITAtech guideline is also missing something else: the definition of what makes a TBM “new”. While opinions from different suppliers vary, Robbins is perfectly clear on this topic: If you are buying a new TBM, then it is a 100% new TBM with only 100% new components. We strongly believe the whole industry should commit to this definition of what makes a TBM “new”, and this definition should be added to the guidelines.
Robbins has throughout the years built up a vast experience in providing the right machine for the right project, whether that means a new or rebuilt machine. As a part of this experience, we are convinced that the life cycle of TBMs should be considered at the earliest stages of the design process. Designing machines with ease of rebuilding in mind ensures that we do not have to start from scratch every time a machine needs work. It also results in time, cost, and energy savings when the time does come to rebuild a machine for a new project. For the industry, this type of perspective is the only economical and sustainable option going forward.
By Sindre Log, Civil Engineer and General Manager for Robbins Norway
What’s New, What’s Next: TBM Equipment for the Mining Industry
TBMs have become well accepted in civil construction tunneling and excavate a high percentage of civil construction projects each year. But each year in the mining industry, far more kilometers of tunnels are excavated for mining purposes than for civil purposes. The amount of tunnels needed for mining operations is staggering.
Some examples from metal mines:
- A large, deep gold mine has over 800 km of tunnels. That is a single mine!
- One small underground metal mine excavates over 13 km of tunnel each year
- In the Sudbury, Ontario mining district, there are over 5000 km of mine tunnels
If tunnels for coal mining were included, the statistics would be even more dramatic. But all these tunnels hold a little-known secret.
The Lack of Mechanized Tunneling
How many of the above thousands of kilometers of mine tunnels do you think have been excavated by TBM? The answer is “Nada”. Compare that to the thousands of kilometers of civil construction tunnels built over the last decades, which required hundreds of TBMs. Why such a great disparity when the objective in both industries is to excavate underground openings as rapidly, economically, and as safely as possible? What are the differences in these industries?
In TBM civil construction, the TBM crew members that know how to make things happen underground, that know how to drive the machines, how to lift heavy components and repair the equipment, how to get the trains in and out to remove the muck and to bring in supplies, are known by a special name. They are known as the “miners”. Yet the only thing they are mining is the muck, no coal, no minerals. But “miners” is a term of reverence for someone who knows how to excavate a tunnel rapidly and efficiently. When a civil tunnel is progressing well, they say “now we’re mining”. (Well, sometimes they also say this sarcastically sometimes when things are going poorly underground.) Why aren’t such talented miners, who know how to make a TBM perform, using their TBMs to excavate thousands of kilometers of mine tunnels each year? What are the differences between civil tunneling and mine tunneling?
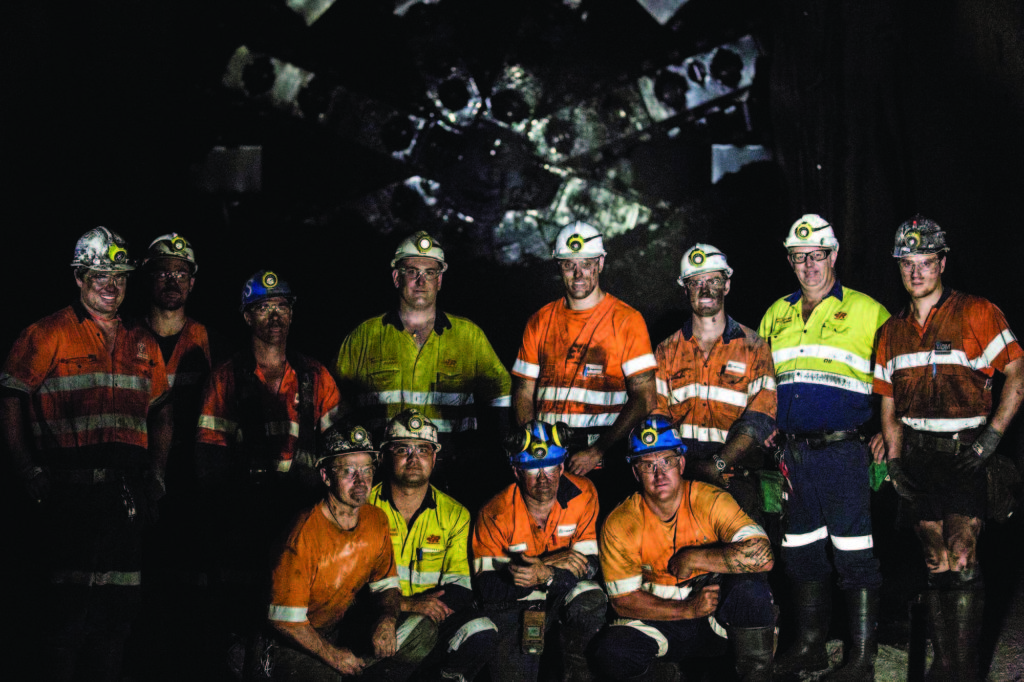
A group of talented miners at Australia’s Anglo American Coal Mine, where a Robbins Crossover TBM excavated two drifts for haulage and personnel access.
TBMs for Civil Tunnels
TBMs have become well accepted for civil tunneling. TBMs designs have become widely adapted for different ground conditions or specialized applications: Hard rock, soft ground, mixed face, pressurized face. Various types of ground support can be installed, according to current geological conditions. More current “hybrid” or “Crossover” TBM designs can handle widely different geological conditions, with the TBM adaptable to cope as conditions change.
Civil tunnels are generally long tunnels, where the efficiency of TBM excavation offsets the longer mobilization and demobilization times. And civil tunnels are generally designed with equipment mobilization/demobilization in mind. Suitable sized shafts are located to allow relatively simple introduction or retrieval of the TBM equipment. The most efficient type of TBM is the full face, rotary type TBM, which produces a circular tunnel profile. The circular profile is nearly universally accepted for civil construction. It is the optimum profile for fluid flow for fresh water, waste water, or hydro tunnels. It is also widely accepted for vehicular civil tunnels that need a flat roadbed. A flat roadway is constructed within the circular tunnel profile and the remainder of the profile within the circle is used for ventilation, services, escapeways, etc.
Some efforts have been made to develop non-circular profile TBMs for the civil sector. These machines include the Mini-Fullfacer, the Mobile Miner, and horseshoe shaped shields with excavator boom or roadheader. Such machines may produce a non-circular profile that is better for that specific job. But usually, there is a penalty in production rate compared to full face, circular TBMs.
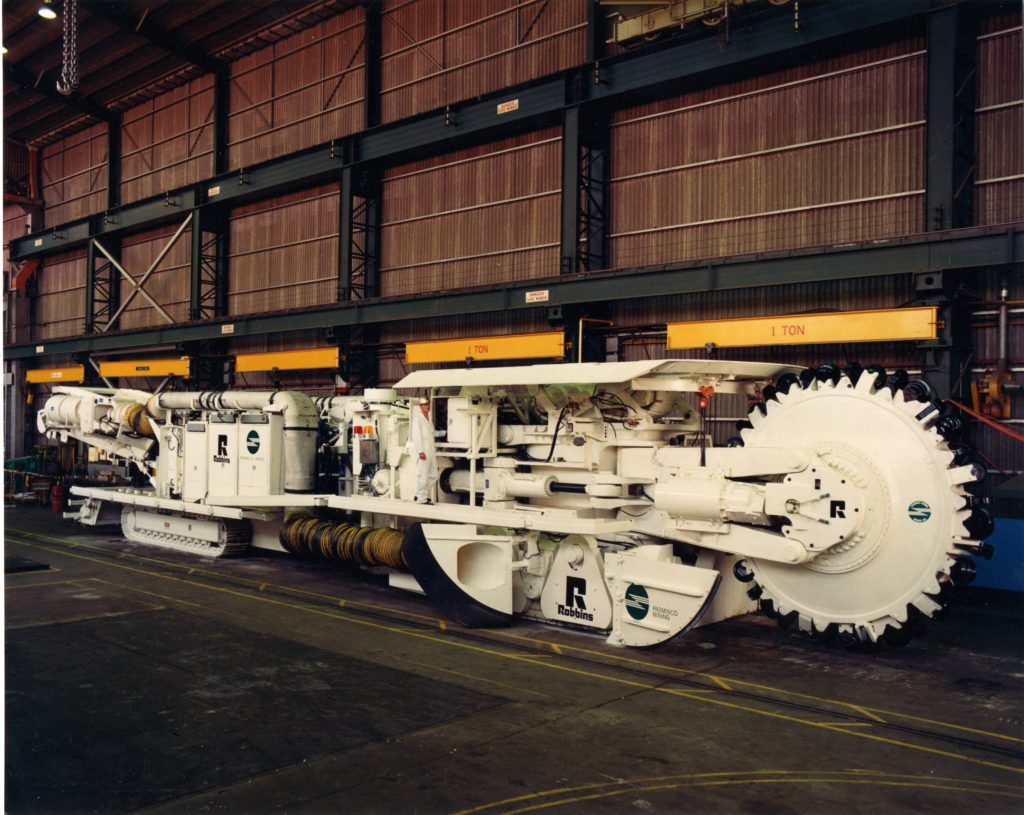
The mobile miner, a non-circular profile TBM developed by Robbins.
TBMs for Mine Tunnels
There have been some notable successes to the application of circular profile “civil type” TBMs for mining projects. Benefits have been lower costs, quicker access, and improved safety. Some examples include:
- Magma Copper, San Manuel Tunnels
- Stillwater Mines (four TBMs used)
- Grosvenor Coal Mine, Two Inclined Access Drifts to the coal seam
However, application of TBMs for mine tunnel construction has remained surprisingly limited. Why is this? Tunnels for mining are often not so long, or a developed in shorter phases, with the excavation front moved from place to place within the mine. TBMs and their constituent components are large and heavy. It is not easy to mobilize a TBM deep underground in a mine at a remote face. Better efforts must be made to make TBM transport and mobilization within the mine practical. This includes considerations for steep ramp roads and other restricted cross sections within the mine.
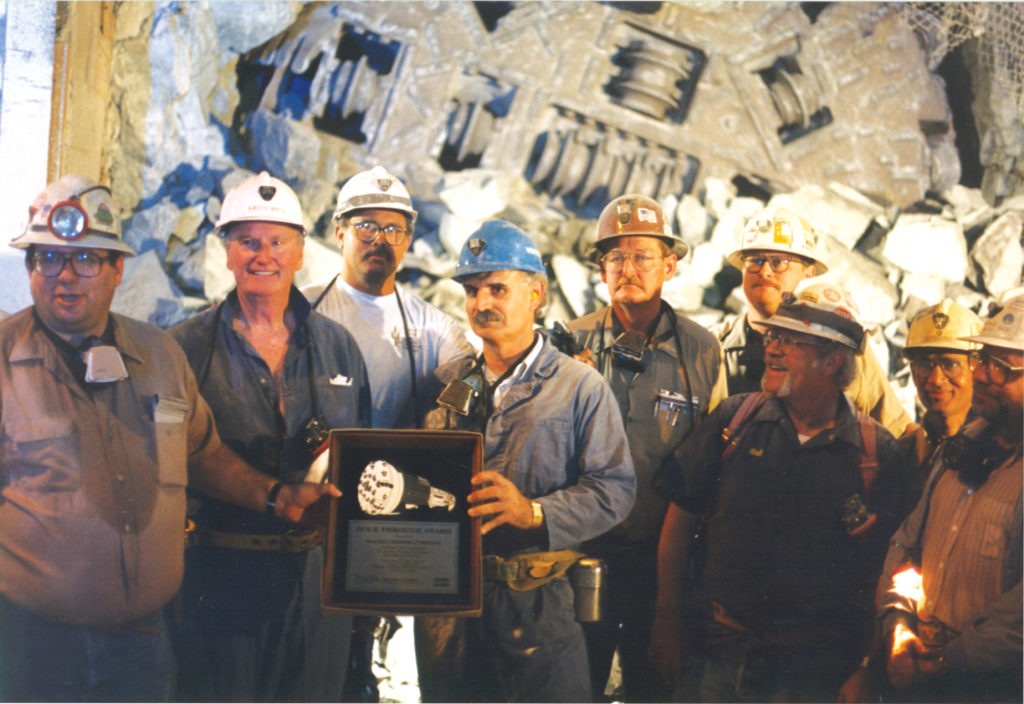
Breakthrough at the Magma Copper Mine in the 1990s.
Efficient TBMs are highly productive, but require a lot of power, ventilation, cooling, and support services. These need to be part of the mining plan so that the TBM has the necessary support and can provide the full benefit. Operating personnel with proper skills are also essential. If a TBM is introduced into a mining environment, either the mine personnel need proper TBM training or motivation, or the TBM drive must be isolated as a “stand alone” operation within the mine and be given proper priority of skilled personnel and the necessary services so the full benefit can be realized.
Mines often do not accept the circular profile produced by the most efficient, full face rotary TBMs. Mine tunnels are usually designed with a flat invert to allow for passage of rubber tired vehicles during the production phase of the mine tunnel. Many efforts have been made to provide TBM type equipment that produces a flat invert. Some have been relatively successful. But generally these machines do not provide the same productivity of efficient, full face circular profile TBMs. Rail bound mining vehicles can be used in the circular tunnel to take advantage of this TBM efficiency. Or, precast invert slabs, poured in place concrete, or partial invert filling can be used in a circular tunnel to provide a flat roadway. The cost/benefits must be analyzed and presented to the industry for a change to occur.
Mining plans often have tunnels with steep gradients and sharp radius curves. On steep gradients (up to 12-15 degrees), the most efficient haulage is usually by belt conveyor. However, the belt system is not effective if there are sharp radius curves. And typical TBM curve ability is limited. Special TBM designs can be made that allow for excavation in sharp curves, but there is a compromise in reduced TBM performance.
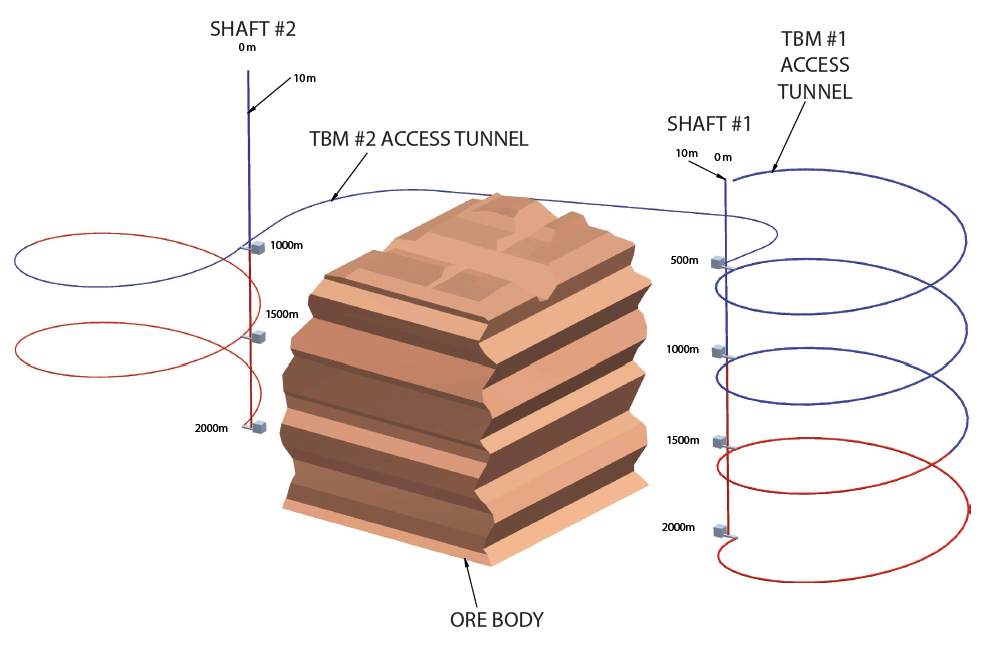
An example of a mine access and haulage plan to reach a deep ore body.
Mines need Versatile TBMs
It seems the mining industry needs the benefits that TBMs can provide. Open pit mines are becoming depleted, and mining activities are reaching deeper. Longer access tunnels are needed. Safety and speed of development are paramount. TBMs can offer these advantages, but have limitations. Special TBMs can be developed that meet special requirements, but usually there is a penalty in reduced TBM performance. The mine planners and the TBM equipment designers must work together at an early stage in the mine planning to determine the optimum compromise between most desirable mine plan, and most beneficial application of TBM equipment. A good partnering approach is necessary in the planning stage, as well as the operating stage, to allow for the most efficient application of TBM equipment to the needs of the mine.
By Dennis Ofiara, Chief Engineer
The Creation of the Crossover
The Real Field Experiences that Resulted in a Versatile Design
This blog is the first in a series called “Hidden Underfoot”, exploring the little-known history and behind-the-scenes happenings of the tunneling industry.
Many technological breakthroughs have been the direct result of the necessity to solve a problem; the creation of the first Crossover TBM was no exception. While much has been written about Central Turkey’s Kargi Kizilirmak Hydroelectric Project, we interviewed Field Service personnel who were there working in the conditions every day to get the untold story behind the genesis of the versatile Crossover TBM.
Behind the Scenes
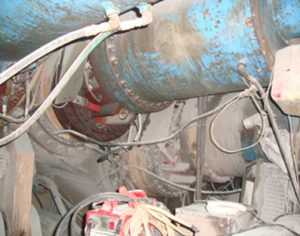
Custom-made gear reducers retrofitted to the motors in the tunnel.
The Kargi HEPP, now complete, generates 470 Gwh of power annually for project owner Statkraft, and supplies an estimated 150,000 homes.
Robbins supplied a 9.84 meter (32 ft) diameter Double Shield TBM and continuous conveyor system to Turkish contractor Gülermak for the project. The machine was to bore an 11.8 km (7.3 mi) headrace tunnel to divert water from the dam to powerhouse. Initial geological reports predicted softer ground for the first 2.5 km (1.6 mi), which would be lined with pre-cast concrete segments. The remainder of the tunnel was to be supported by a combination of shotcrete, rock bolts, and wire mesh in more competent rock.
The project became arduous soon after startup, when the machine encountered blocky rock, sand, and clays that were not initially predicted. “We realized modifications needed to be made as soon as we started experiencing flowing materials and squeezing ground,” said Glen Maynard, Robbins Site Manager, who worked on the project throughout the challenging conditions. Maynard’s prognosis was apt, as 80 meters (262 ft) into the bore the TBM became trapped in a section of collapsed ground. “The machine faced serious blockages,” added Maynard, “there was no one fix, each problem needed its own solution. Thrust, cutterhead, and ground support all needed adjustment.” The machine was freed but continued to struggle, requiring not just one but seven bypass tunnels to free it each time it encountered collapsing ground.
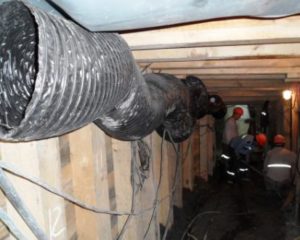
Construction of one of several bypass tunnels at Kargi.
Concerns that the machine would need to be buried were quickly mollified. Robbins President Lok Home told the Kargi team to build a “wish list” of all the materials they would need to modify the machine and get it moving again. Robbins and Gülermak worked together to determine what changes would need to be made, and parts were shipped to the jobsite as quickly as possible. This of course, was not the first time a machine had required modifications while in the tunnel, and Robbins engineers were able to pull from past projects in order to know what needed to be done.
In-Tunnel Effort
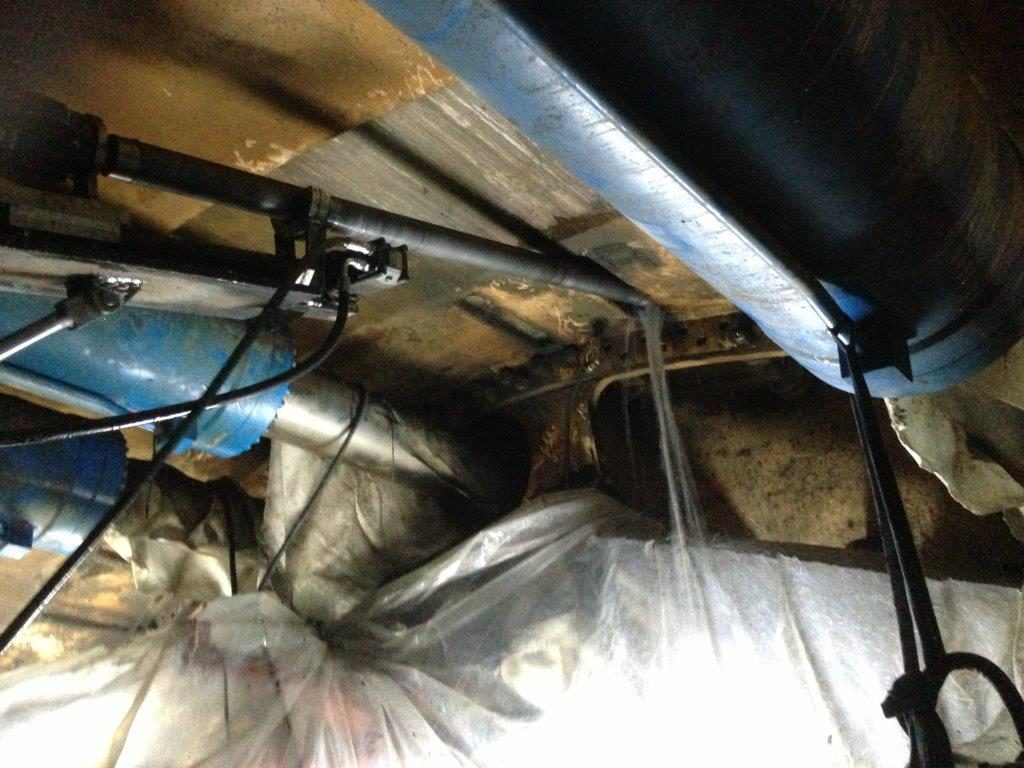
Canopy drill installed in the tunnel.
The contractor, with the assistance of the field service team, installed a Robbins custom-built canopy drill and positioner to allow pipe tube support installation through the forward shield. This allowed drilling with a distance of up to 10 m (33 ft) ahead of the cutterhead while 90 mm (3.5 in) diameter pipe tubes provided extra support across the top 120 to 140 degrees at the tunnel crown. Injection of resins and grout protected against collapse at the crown while excavating through soft ground. “There were a lot of risks to the modified machine, but we gave comprehensive instructions on how to operate it to the contractor,” said Maynard. “The contractor team was open to change and had great cooperation. They were a really hard working team.”
To further mitigate the effects of squeezing ground or collapses, custom-made gear reducers were ordered and retrofitted to the cutterhead motors. They were installed between the drive motor and the primary two-stage planetary gearboxes. When the machine encountered loose or squeezing ground the reducers were engaged, which resulted in a reduction in cutterhead RPM and a doubling of the available torque. The net effect of the modifications allowed the Double Shield TBM to operate much like an Earth Pressure Balance Machine in fault zones and squeezing ground with high torque and low RPM—these methods effectively kept the machine from getting stuck. In addition, short stroke thrust jacks were installed between the normal auxiliary thrust to double total thrust capabilities.
The results of the modifications led to astonishing results. An advance rate of 600 m (1,986 ft) in one month was achieved in March 2013 and a project best of approximately 723 m (2,372 ft) was achieved in spring 2014, including a daily best of 39.6 m (130 ft) in April 2014. The TBM bored 7.8 km (4.8 mi) of the tunnel in total, making its final breakthrough in July 2014. The remainder was excavated by drill and blast—of which it is notable that the modified TBM achieved advance rates more than twice that of the traditional mining operation. “It was enjoyable to find a way to overcome this challenge,” added Maynard.
Mixed Ground Legacy
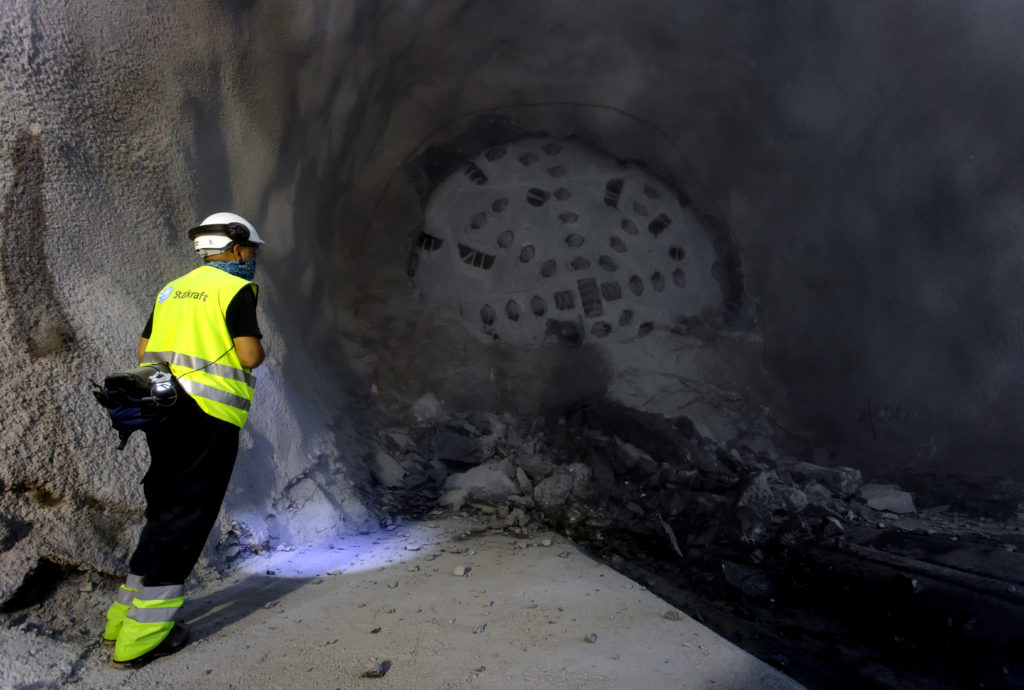
Successful breakthrough in July 2014.
A project that had started out as a disheartening one transformed into one that encouraged others. The insights gained by implementing changes to the Kargi machine were quickly applied to other projects facing mixed ground conditions that would otherwise require multiple tunneling machines. From these creative solutions came the design of a new line of Robbins dual-mode machines—TBMs that contain features of two machine types—termed Crossovers. Everything from multi-speed gearboxes to canopy drills, and emergency thrust are regular features of Crossover machines, and they owe their genesis to the hard work involved at Kargi HEPP.
From Risk Aversion to Risk Reduction: How Elon Musk could usher in a New Era of Tunnel Boring
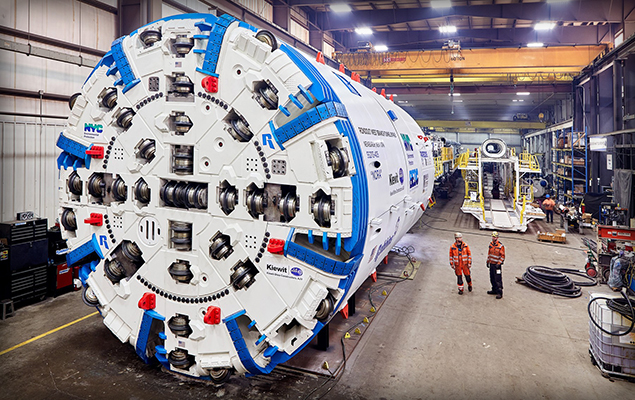
Tunnel boring machines like the one here, for New York’s Delaware Aqueduct Repair, are turning risk aversion on its head.
It has been some time since I have written on the Robbins blog page, but I am inspired to do so by the announcement that Elon Musk is entering our business—the tunnel boring business. It is great to see people with a vision of an improved world enter our industry. I agree with Musk that the advance rate of tunnels can be significantly improved if development money comes into the industry. Development money in tunneling, however, is at best minimal and is more often essentially nonexistent. Nearly all tunnels are heavily specified to avoid risk taking by owners (therefore discouraging new development). Nearly all tunnels go to the low bidder and low bidders try to buy the TBMs at the lowest price; a further discouragement of development. The industry has therefore been slow to improve advance rates, but with Musk bringing the issue into the spotlight, perhaps things will change.
Risk Aversion and How to Reverse it
There are some exceptions to this practice of risk aversion for new technology, and one is the Delaware Aqueduct Repair. This tunnel corrects heavy water leakage occurring from the 1940’s-built aqueduct tunnel for New York City. We are just completing Factory Acceptance in our plant in Solon, Ohio of this unique Single Shield TBM. The tunnel is at significant depth (approximately 300 m / 900 ft) with the distinct possibility of encountering very high water pressure (up to 30 bars). The contractor JV of Kiewit/Shea have shown their willingness to move forward with several new developments for this project. The concept of grouting off high water pressure as the primary means to allow advance in such conditions, rather than use an EPB or Slurry TBM, is in my view a significant step forward for our industry. Granted there have been halfway attempts with a combination of grouting and pressurized tunneling at recent projects like the Arrowhead Tunnels and Lake Mead Intake No. 3, but these have come at high cost and sometimes long delays. The Delaware Aqueduct TBM, by contrast, is designed to hold up to 30 bars of pressure while grouting occurs. Boring and cutter changes are done in atmospheric pressure.
Chemical grouting and grouting technology in general have advanced multifold in recent years, and it is commendable to see it used extensively on several aspects of the Delaware Aqueduct Project. It’s a great example of what can be done when a contractor is willing to use new technology to address potential risks—it appears it can actually reduce risk in the long run. It is a great honor to be working with the capable Kiewit/Shea JV team to be a part of advancing technology.
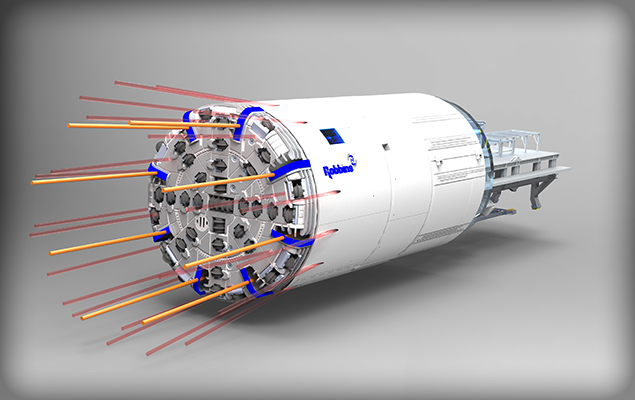
The enhanced probe drilling and grouting capabilities as seen in trajectories (orange and red) on the Delaware Aqueduct TBM 3D model.
Areas Ripe for Change
The Delaware Aqueduct Repair project is a flagship project for what I hope will become more common in the industry: instead of low bidding with the cheapest possible machine, offering a reasonable bid with a specialized TBM that has a higher initial investment, but ultimately a lower cost overall. The project’s use of technology is wide-reaching, particularly atmospheric cutter changes and chemical grouting, which have the potential to reduce downtime and increase safety. I do not see the future of rock tunneling under high water pressure being left to divers to change cutters and repair the cutterhead. We all know it is not cost effective to send divers to work in confined spaces over 10 bars. It should be noted that the long-duration Hallandsås Tunnel, for example, finished the majority of its TBM advance by relying on effectively this technique of grouting and advance after failing with a Slurry System. There are lots of tunnels to be built with above 10 bars pressure that will use this technology. The industry needs to automate cutter and bit changes as much as possible, and increase the integration of chemical grouting in tunneling.
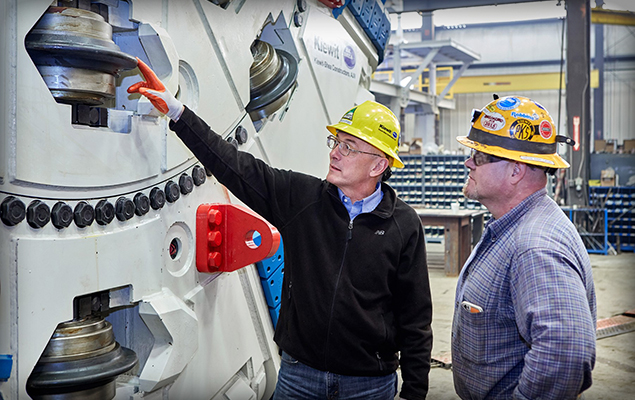
Niels Kofoed and Danny Smith of contractor Kiewit examine the cutterhead and cutters of the Delaware Aqueduct TBM. The machine can bore and cutters can be changed at atmospheric pressure.
Certainly there are many areas for advancement in our industry, and major public figures like Musk drawing attention to it is ultimately a good thing. After all, getting the general public to think about solving traffic by going underground is no easy feat. Even more so, getting the tunneling industry to think about its own risk-averse practices has a big potential benefit. Hopefully all of this attention will result in more tunnels, more business, and better infrastructure. Musk’s willingness to take a risk aimed at making the underground construction industry potentially faster and more stable is a good bet to take.